Testing Hardness: Methods and Applications in Materials
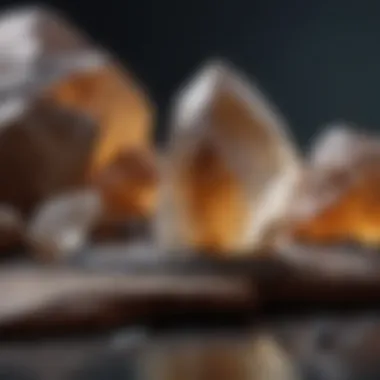
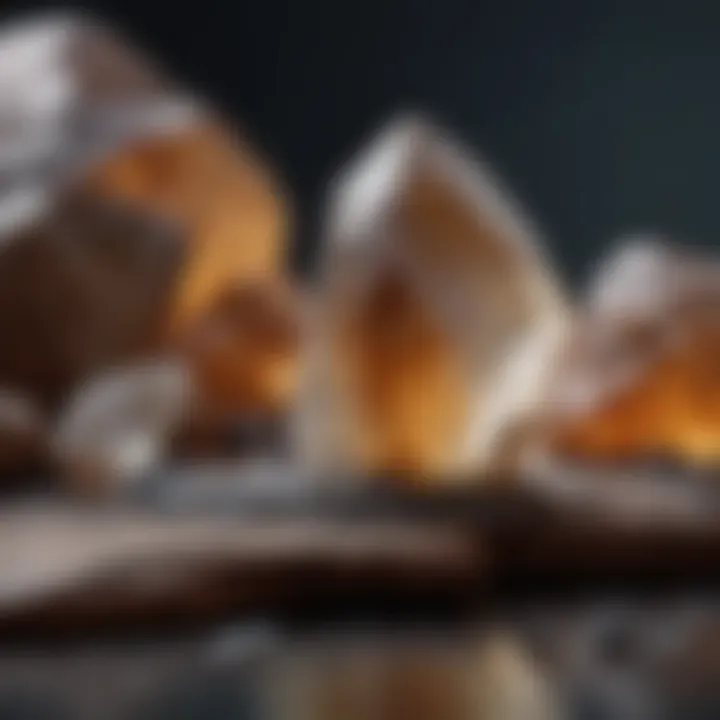
Intro
Understanding the hardness of materials plays a pivotal role in numerous scientific and industrial applications. This encompasses everything from metallurgy to geology and aids in making informed decisions in areas such as manufacturing, construction, and even gemology. Hardness tests help us determine how materials will react under stress or abrasion, which in turn informs their suitability for various uses. By gearing our focus towards methods like Mohs, Vickers, and Brinell scales, we aim to unravel the intricate landscape of hardness testing.
Hardness is not merely a number; it tells a story about the material's properties and potential applications. Whether it's the steel used in construction or a gemstone set in jewelry, knowing the hardness aids in making educated choices for any endeavor. As we delve deeper into the methods and applications associated with hardness testing, keep an eye on the implications of these metrics in real-world scenarios.
Understanding Hardness
Hardness is more than just a measure of how resistant a material is to scratching or denting; it is a key concept that influences decisions across various fields, especially material science and geology. The ways in which we assess and understand hardness can greatly affect material selection, quality control, and even a collector's ability to evaluate specimens. In essence, understanding hardness allows professionals and hobbyists alike, particularly rock and fossil collectors, to appreciate the intrinsic properties of the materials they are dealing with.
A solid grasp of hardness testing presents several benefits. Firstly, it enables informed choices about materials for specific applications. For instance, a collector might prefer a harder mineral when choosing specimens for an exhibit to ensure durability and aesthetic appeal. Secondly, it aids in the valuation of materials; harder gemstones, for example, often command higher prices in the market. Additionally, it fosters a deeper appreciation for the natural world—recognizing why some rocks withstand erosion better than others can enhance a collector's connection to their specimens.
Instrumental to this understanding are the various methods used to measure hardness. Familiarity with these techniques allows collectors and scientists to communicate effectively about the properties of their materials. Moreover, knowledge of hardness implications can lead to more strategic decisions regarding preservation and maintenance of collections, ultimately extending the lifespan of the pieces in question.
Definition of Hardness
The term "hardness" refers to a material's resistance to deformation, scratching, or abrasion. It's a relative measure, signifying how one material compares to another—this relativity is evident in multiple testing scales. At its core, hardness can be described in geometric or mechanical terms, often seen as how deep a given object can penetrate a surface. For instance, diamonds are rated as one of the hardest materials known, while talc ranks low on the scale, representing the variety found in the mineral kingdom.
This definition extends beyond just rocks and minerals. In engineering contexts, metals are often tested for hardness to assess their suitability for certain conditions. Knowing how groups of materials interact under stress can dictate design choices in construction or manufacturing.
Importance in Material Science
Hardness testing stands at the forefront of material science due to its inherent link to several physical properties. It correlates with attributes like elasticity and tensile strength, offering insights into how a material will respond under various forces. The relationship between hardness and other properties serves as a critical guideline, particularly in the formulation of new alloys or composites.
Furthermore, as material requirements evolve with advancing technologies, understanding hardness becomes essential. The shift towards lightweight yet durable materials in industries such as aerospace and automotive necessitates that scientists and engineers continually refine their testing methods and incorporate new findings into their work. This ongoing evolution pushes the boundaries of what is achievable in terms of material performance and safety.
Applications in Various Industries
Hardness has vast applications across various industries, and their implications can affect everything from production processes to final product durability. Here are a few notable examples:
- Geology: Hardness testing determines mineral identification and helps collectors understand how materials will weather.
- Manufacturing: Industries use hardness measurements to ensure compliance with standards, influencing everything from automotive parts to consumer goods.
- Construction: Knowledge about the hardness of stones and aggregates influences building material choice, which can affect structural integrity.
"Understanding the hardness of materials helps bridge the gap between theoretical knowledge and practical application across diverse fields."
- Jewelry: In gemology, hardness tests aid in determining the value of gemstones, important for both collectors and appraisers.
- Electronics: Hardness assessments are significant for selecting materials that endure stress and heat, which is critical for electronic devices.
Collectively, these applications highlight how critical it is to delve into the nuances of hardness testing. Whether for academic purposes or personal collecting passions, the implications of hardness resonate throughout the fabric of material interactions.
Historical Context
Understanding the historical context of hardness testing is crucial for grasping its significance and evolution within material science and geology. Rather than considering hardness testing as a recent invention, delving into its origins and the development of various scales enriches our comprehension of its applications today. The journey from early trials and determination methods to the precise advancements seen nowadays reveals much about how our understanding of materials has evolved. This section serves to illuminate the path taken by scientists and researchers that shaped the methods we currently utilize, highlighting the continual need for reliable and efficient testing protocols.
Origins of Hardness Testing
The concept of testing hardness is not entirely modern. In fact, the roots of this practice can be traced back to ancient civilizations. The earliest forms of hardness testing involved simple, practical observations rather than structured methodologies. For example, the Greeks and Romans would have intuitively compared materials by scratching one against the other to determine relative hardness—a rudimentary and somewhat imprecise method, but effective in its own right.
As human curiosity about the physical properties of materials grew, so did the desire for more systematic approaches. By the 19th century, scientists such as Friedrich Mohs began formalizing hardness testing. Mohs introduced his scale in 1812, creating a reference system that defined hardness in a relative way—using ten minerals as benchmarks, with talc being the softest and diamond the hardest.
This shift marked a significant progression in how hardness was perceived and assessed, laying the groundwork for future testing endeavors. An understanding of hardness began to underpin various scientific and industrial practices, guiding decisions in engineering, geology, and manufacturing.
Evolution of Hardness Scales
The evolution of hardness scales is a testament to the scientific community’s ongoing commitment to accuracy and innovation in material testing. After Mohs’ introduction of his scale, several other methods emerged to address limitations and cater to different materials.
For instance, in the early 20th century, the Vickers hardness test was developed. It introduced a more quantitative approach, allowing for minute measurements and suitable for testing various metals and ceramics. This method employed a diamond indenter to produce an impression, measuring the diagonal of the indentation to determine hardness—a dramatic transformation from earlier methods.
Meanwhile, the Brinell test, also developed in the same era, made its mark by specifically evaluating larger materials, such as metals, with a steel or carbide ball indenter. Such developments not only catered to a wider range of materials but also fed the industry’s need for standardized testing metrics.
Ultimately, these advancements in hardness testing methods reflect an intricate dance of scientific inquiry and practical necessity, making them indispensable in today’s landscapes of materials science and industry. By studying the origin and evolution of hardness testing, collectors and industry professionals alike can appreciate not just the methods themselves but the rich history that continues to inform current practices.
Hardness Testing Methods Overview
Understanding the various hardness testing methods is crucial in the fields of material science and geology. These methods provide insights into how materials respond to stress and wear, significantly affecting their usability in practical applications. For rock and fossil collectors, knowing how to assess hardness can guide decisions about specimen value and care. Additionally, recognizing the strengths and weaknesses of different testing techniques helps inform which method is best suited for a specific material or context.
Moreover, employing appropriate hardness testing methods can prevent misclassification of invaluable specimens. For instance, the hardness of minerals can give clues about their origin and formation processes. It also plays a vital role in predictive maintenance for industrial applications, ensuring materials can withstand operational demands without failure. Hence, grasping these methods isn't just academic; it's a necessary skill for anyone deeply involved with materials.
Indentation Methods
Indentation methods remain a backbone of hardness testing, relying on creating an impression of a standardized load on the material. The most recognizable techniques in this category include the Vickers and Knoop hardness tests. In these tests, a diamond indenter is used to press into the material. The resulting indent's dimensions can be measured to calculate hardness values. This approach excels in providing accurate and repeatable results, making it particularly suitable for small and brittle samples.
Using indentation methods permits high precision, especially when one needs to measure the hardness of thin films or fragile minerals. However, these methods also come with some drawbacks, like the potential for surface damage or alteration, which could influence the test's outcome. Therefore, it's essential to consider both the nature of the material and the specific requirements of the test.
Key Points:
- High precision for small samples
- Suitable for various materials
- Potential for sample alteration
Rebound Hardness Tests
Rebound hardness tests, by contrast, measure the energy lost when a mass rebounds from a material surface. Here, instruments like the Shore and Leeb hardness testers are used to quantify this energy dependency. These tests provide rapid measurements without needing significant preparation of the material, which is beneficial in field conditions like geological surveys.
Collectors often find rebound tests useful where quick assessments matter—think of outdoor adventures or museum collections where downtime might lead to missed opportunities. However, it's worth mentioning that results can sometimes be influenced by surface conditions or the sample's homogeneity. Thus, an experienced tester should know how to interpret the results with careful context in mind.
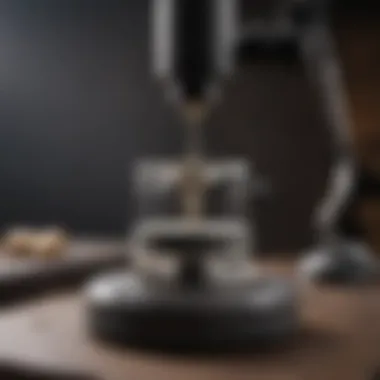
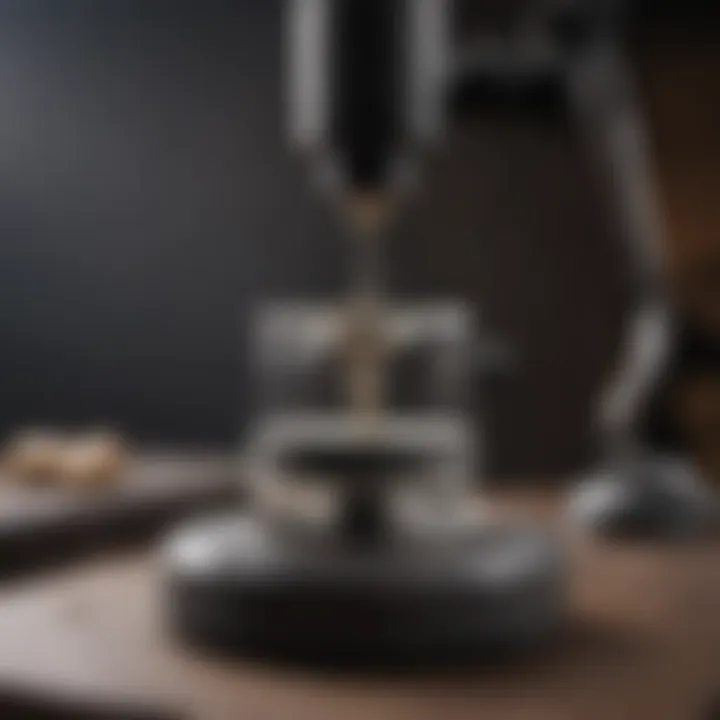
Advantages:
- Quick and easy to perform
- Minimal surface preparation required
- Effective in field applications
Penetration Testing
Penetration testing, often employed in both natural and synthetic materials, involves measuring how deeply a pointed object can penetrate a given surface under specific conditions. Rock and fossil collectors utilize this method to assess hardness, particularly in softer materials or specimens where conservation is paramount.
This technique is often simpler and less equipment-intensive compared to indentation tests. While it lacks some precision, its non-destructive nature adds a layer of appeal for those seeking to preserve the integrity of their collection. It's a practical choice when collectors want to evaluate a broader range of specimens without altering them significantly.
Considerations:
- Less precise but non-destructive
- Useful for a variety of materials
- Requires careful handling to maintain specimen integrity
By understanding these methods and their contextual applications, collectors, scientists, and engineers can make informed decisions, enhancing both academic knowledge and practical approaches to working with materials.
Mohs Hardness Scale
The Mohs Hardness Scale holds a pivotal place in the realm of material science, especially relevant for geologists and mineralogists. It serves as a simple yet effective way to assess the scratch resistance of various minerals through a comparative method, allowing collectors to prioritize their specimens and understand their physical characteristics. Characteristics such as abrasiveness, durability, and usability in specific applications can be gauged using this scale, enhancing its importance.
Origin of the Mohs Scale
Developed in 1812 by the German geologist Friedrich Mohs, the scale originated from a desire to create a straightforward classification system based on scratch tests. Mohs employed ten common minerals as reference points – from talc, the softest, to diamond, the hardest. This foundational approach facilitated easier communication among scientists, creating a standardized set of references for mineral hardness. The establishment of this scale not only allowed for enhanced classification but also laid the groundwork for more sophisticated hardness testing methods in subsequent years.
Methodology of Use
Utilizing the Mohs Hardness Scale involves simple scratch tests, which require a selection of the reference minerals. The steps are straightforward:
- Gather Minerals: Collect the mineral of interest and several minerals from the Mohs scale, each representing a different hardness level.
- Scratching Test: Gradually scratch the sample using the reference minerals, starting with the softest. If the test mineral leaves a scratch on your specimen, it is softer than the mineral being tested.
- Comparison: Continue this process until no scratch is made; the last mineral that scratched is indicative of the test mineral's hardness. Each mineral on the scale is assigned a designated hardness ranging from 1 to 10.
This method is often favored for its simplicity, especially in fieldwork, where equipment may be limited, allowing collectors to test their specimens directly.
Interpreting Results
Once the scratch test is complete, the next step is interpreting the results to ensure practical understanding. Each mineral's hardness is assigned a number:
- Talc - 1
- Gypsum - 2
- Calcite - 3
- Fluorite - 4
- Apatite - 5
- Feldspar - 6
- Quartz - 7
- Topaz - 8
- Corundum - 9
- Diamond - 10
In this scoring system, knowing the hardness can inform collectors about their specimen's durability and potential applications. For example, a mineral graded at 5 means it can easily be scratched by a 6 but can scratch those rated below it; thus, a careful choice of storage and handling is crucial. Minerals such as quartz, scoring a 7, are more resilient in both natural and decorative applications compared to softer minerals.
Understanding the Mohs Hardness Scale is essential for any rock or fossil enthusiast, as it provides key insights into material properties that affect both collection and valuation.
By utilizing the Mohs Hardness Scale effectively, rock and fossil collectors can make informed decisions regarding their acquisitions and understand the implications of their specimens' hardness, enhancing both the enjoyment and practical usage of their collections.
Vickers Hardness Test
The Vickers hardness test stands out as a pivotal method for measuring the hardness of a material. Its influence extends across various sectors, especially for professionals in material science and geology, making it essential knowledge for anyone involved with rocks and minerals. The hallmark of the Vickers test is its versatility; it is applicable to a wide range of materials, from soft copper alloys to the hardest ceramics. This reliability stems from its ability to produce accurate and repeatable measurements, which are crucial when assessing the quality and characteristics of a sample. Moreover, as hardness correlates directly with wear resistance and durability, understanding how to effectively use the Vickers test can greatly inform decisions about material selection in various applications.
Procedure Overview
The procedure for conducting a Vickers hardness test is methodical and straightforward, involving several key steps:
- Sample Preparation: The surface of the sample must be polished to ensure that the measurements are not influenced by surface imperfections or contaminations.
- Indentation Creation: A diamond pyramid indenter, characterized by its square base and equal sloping sides, is pressed into the sample under a specific load for a predetermined duration. The load typically ranges from 1 kg to 100 kg.
- Measurement: After the indenter is removed, the diagonals of the resultant indentation are measured using a microscope. The average of these diagonals is calculated to determine the diameter.
Through this inspection, the Vickers test not only determines hardness but also helps in understanding the material's structural integrity and performance under stress.
Advantages and Limitations
Understanding the strengths and drawbacks of the Vickers hardness test is crucial for users. Here are some key points to note:
Advantages:
- Wide Applicability: This test can be used on almost any type of material, making it versatile for various applications.
- Accurate Reading: Due to the precise nature of the diamond indenter and the mathematical calculations, the results are highly accurate.
- Non-destructive Options: With minimal force, the test can be performed in a way that significantly reduces damage to the test specimen.
Limitations:
- Time-Consuming: Preparing samples and the measurement process can take longer compared to other hardness tests, especially if precision is demanded.
- Equipment Cost: The initial setup for the Vickers test can be relatively expensive due to the speciality of the equipment.
- Surface Conditions: As with most hardness tests, surface conditions greatly affect the outcome; therefore, any inconsistency can lead to misleading results.
Overall, the Vickers hardness test is a robust means of evaluating material properties, although it requires careful execution and an understanding of its context in material selection. In the world of rock and fossil collectors, having a solid grasp of such testing methods bolsters one's capability to analyze and value collections accurately.
"The precision with which the Vickers hardness test operates underscores its relevance in material science, offering insights far beyond just numbers."
For a deeper dive into hardness testing methods and their specific applications, resources like Wikipedia and Britannica can provide extensive background and updated information.
Brinell Hardness Testing
Brinell hardness testing plays an essential role in the world of material science and testing, particularly when it concerns metals. Developed by J.A. Brinell in 1885, this method uses a hard spherical indenter to measure the hardness of materials, providing a quantifiable value that reflects a material's resistance to deformation. The significance of Brinell testing lies not only in its historical context but also in its ability to furnish reliable data across diverse applications, including metallurgy, manufacturing, and the geosciences.
One of the key benefits of employing Brinell hardness testing is its ability to gauge the hardness of materials with larger grains. This is particularly relevant to rock and fossil collectors, as many geological specimens do not have the uniformity seen in industrial metals. Brinell utilizes a ball indenter and applies a predetermined load, subsequently measuring the indentation size left in the target surface. Through the diameter of this indentation, hardness values can be calculated, which can then be compared with established hardness scales.
However, considerations must be taken into account when using this method. The surface quality, cleaning procedures, and even the type of indenter material can all impact test results. Brinell testing is not suited for materials that are too hard or too brittle, which is where other methods like Vickers or Rockwell might be more applicable. Knowing when to use Brinell is a critical skill for professionals in fields like geology or metallurgy, ensuring accurate assessments that can guide material selection and application.
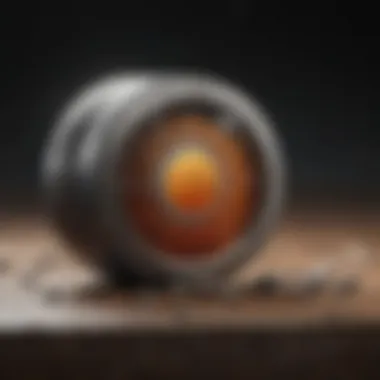
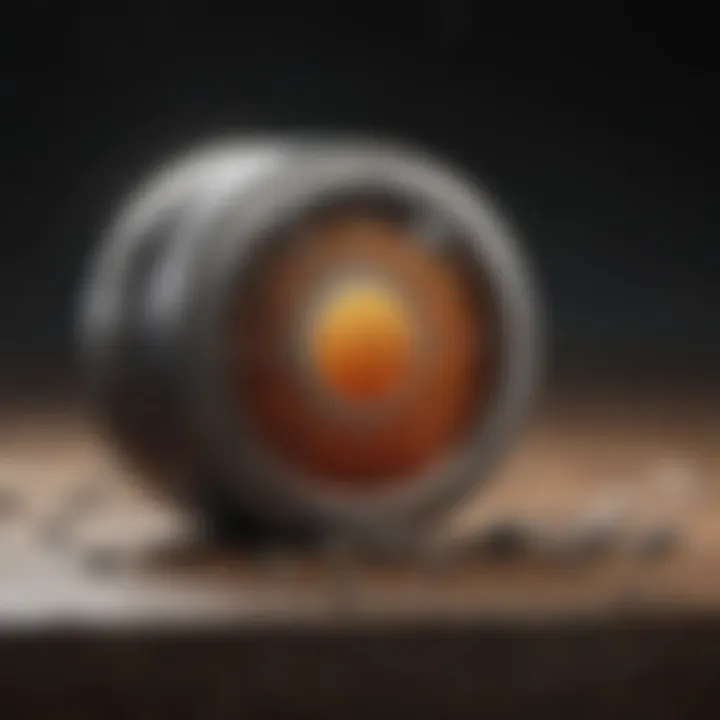
Testing Equipment
The equipment necessary for Brinell hardness testing includes several key components that work in unison to deliver precise measurements. The primary device you will encounter is the hardness tester, which is designed to apply uniform pressure while providing a controlled environment for testing.
- Indenter Type: The indenter is typically made of hardened steel or carbide, shaped as a sphere. The diameter of this sphere is commonly 10mm, though various sizes are available for different materials.
- Load Application Mechanism: Depending on the material being tested, loads can range from 500 kgf to 3000 kgf, requiring a reliable load application system. This might be hydraulic or mechanical, so attention to detail during the setup is vital.
- Measurement Tools: After the load is applied and the indenter is removed, you'll need precise measurement tools such as a microscope or measuring caliper to accurately assess the indentation size. Most modern testers include built-in measurement systems that simplify this step.
"The Brinell hardness tester is like a balance scale. Without the right setup and technique, results will tip far from the truth."
Interpreting Hardness Values
Understanding how to interpret the values gained from Brinell testing is crucial for applications in geology and material science. The results are typically reported in Brinell Hardness Number (BHN), calculated using the formula:
where:
- P = load in kgf
- D = diameter of the indenter in mm
- d = diameter of the indentation in mm
The Brinell hardness value offers insights into a material's mechanical properties. For instance, a higher BHN indicates increased hardness and generally correlates with better wear resistance and strength. However, keep in mind that hardness values should never be viewed in isolation. Contextual factors like grain size, purity, and even environmental conditions can all influence hardness measurements.
This method produces larger indentations, which could lead to some material loss but provides a broader view of the material's hardness, particularly when considering heterogeneous or coarse-grained samples that are typical in geological studies. Thus, interpreting Brinell hardness values is not just a mechanical exercise but also a crucial interpretative skill that helps collectors and professionals understand the usability and characteristics of various rock and mineral specimens.
Rock Hardness Testing Techniques
Rock hardness testing is a vital aspect of geology and material science. It serves as a window into the durability and behavior of a variety of rock types, providing valuable insights for collectors and professionals alike. Understanding the hardness of geological specimens helps in assessing their overall quality, which can significantly impact their desirability and, consequently, their market value. Moreover, knowledge of hardness can guide collectors in choosing the proper methods for field work and laboratory studies.
Field Tests for Collectors
Field tests offer collectors a straightforward way to gauge hardness without requiring extensive laboratory resources. These tests emphasize convenient and rapid measurements, making them ideal for on-the-spot evaluations of rock qualities in the terrain. Some simple techniques include:
- Scratch Test: Using a known mineral or object, like a fingernail or a metallic key, collectors can scratch the rock surface. The ability of the rock to resist scratches gives immediate clues about its hardness. For example, talc, which is rated 1 on the Mohs scale, can easily be scraped, while quartz, scoring a 7, cannot.
- Rock Hammer: A collector can tap a rock with a hammer. If the sound is dull, it showcases less hardness compared to one that produces a ringing sound, indicating a tougher mineral composition.
- Moisture Absorption: Observing how quickly a rock absorbs water can also hint at its hardness. Softer rocks generally absorb moisture faster than harder, more dense rocks.
Field tests are beneficial for collectors who may lack lab facilities, providing them a practical method to categorize and assess their finds.
Laboratory Methods for Rocks and Minerals
Laboratory methods bring precision to hardness testing and are essential for deep analysis. Here, more sophisticated equipment and techniques allow for the assessment of rock samples with accuracy. Common laboratory methods include:
- Vickers Hardness Test: This involves applying a known force on a diamond indenter, creating a precise indent in the rock. The size of the indent is measured under a microscope, providing a direct reading of the hardness. It is particularly effective for harder minerals and rocks, where fine distinctions are necessary.
- Brinell Hardness Test: Though not often used for rocks, this method can be applied when a larger, softer specimen needs testing. A steel ball is pressed into the sample, measuring the indentation made, which correlates to hardness.
- Mohs Scale Usage: While the scratch test operates in the field, laboratory use often employs a detailed understanding of the Mohs scale, where a sequence of minerals serves as comparatives. By systematically scratching samples against known minerals, a clearer understanding of hardness comes to light.
- Ultrasonic Testing: This advanced technique measures the speed of sound waves as they travel through a material. Because sound waves travel faster in harder materials, this can indicate hardness levels with great accuracy.
Utilizing laboratory methods enables precise, repeatable results, enhancing knowledge about rock materials while assisting in geology education, research, and industry applications.
"Knowing the hardness of rocks often separates the dabbler from the serious collector, providing insights that simply scratching cannot achieve."
In culmination, understanding both field and laboratory methods for hardness testing creates a well-rounded approach for rock and mineral collectors. Collectors can effectively navigate both environments, armed with techniques that range from simple to sophisticated, establishing confidence in their assessments.
Factors Affecting Hardness Testing
Understanding the factors that impact hardness testing is crucial for those engaged in material science and geology. When evaluating the hardness of materials, several elements can significantly influence the results. These elements range from the composition of the material itself to the environmental conditions under which the testing is conducted.
By grasping these factors, rock and fossil collectors—alongside professionals—can make more informed decisions regarding material properties, durability, and suitability for specific applications. In essence, these insights aid in ensuring the reliability of hardness values and their subsequent interpretation.
Material Composition
The makeup of a material is arguably one of the most critical factors affecting hardness testing. The chemical and physical characteristics of a material play a vital role in determining how it will react under stress. For instance, metals like steel, due to their crystalline structures and alloying elements, exhibit varying levels of hardness depending on how they are formed, heat-treated, or treated with certain alloying elements such as carbon.
Some key considerations regarding material composition include:
- Alloying elements: The addition of elements such as chromium, molybdenum, or nickel can enhance hardness through mechanisms like solid solution strengthening.
- Grain size: Finer grains typically improve hardness and strength due to the increased number of grain boundaries, which serve as barriers to dislocation movement.
- Phase transformations: In materials that can exist in different phases, such as metals transitioning from austenite to martensite, the resulting phase can profoundly affect hardness.
In the context of geological specimens, hardness can be influenced by not just the minerals present but how they bond together. For collectors, identifying these factors can enhance their understanding of the material’s durability and desirability.
Environmental Conditions
The environment in which hardness testing occurs can also introduce variables that can skew test results. Such conditions may include temperature, humidity, and even ambient pressure.
For example:
- Temperature: Many materials exhibit changes in hardness with temperature fluctuations. Generally, increased temperatures can lead to a decrease in hardness—in glass, this effect is pronounced as it softens at high temperatures.
- Humidity: In certain materials, particularly those susceptible to moisture, humidity can affect the integrity of the substance being tested. For instance, wood can swell or warp with high humidity levels, altering the result from a hardness test.
"Understanding the interplay between material composition and environmental conditions is key to accurate hardness testing and interpretation."
Interpreting Hardness Data
Understanding how to interpret hardness data is pivotal in the fields of material science and geology. It not only shapes the way we classify and assess materials but also influences how we use them in various applications. Therefore, having a firm grasp of hardness data is essential for anyone involved in these fields, especially rock and fossil collectors who want to make informed decisions about their collections.
Comparative Analysis Across Scales
When comparing hardness values, one must navigate different scales, each with its unique metric and applications. For instance, the Mohs scale, which ranks minerals based on their ability to scratch one another, operates on a non-linear index. A diamond sits at the top at 10, while talc rests at 1. This scale provides a straightforward method for geologists to categorize minerals in the field, making it particularly useful during exploration.
However, one must tread carefully when interpreting hardness data across different scales. Not all scales equate directly—Vickers and Brinell scales provide numerical values that can confuse someone accustomed to the Mohs scale. A Vickers hardness value of 1000 can correspond with a Brinell hardness number that differs significantly. To ensure accuracy, a thorough comparative analysis is essential, considering factors like the test method and the material being assessed.
Moreover, it’s practical to utilize conversion charts or mathematical equations specifically designed to relate these scales. Not everybody has these memorized, though; familiarity can come with experience and experimentation.
"In the marketplace of rocks and minerals, knowing their hardness is like having insider knowledge; it can tilt the trade in your favor."
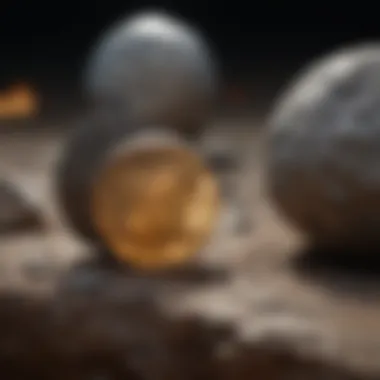
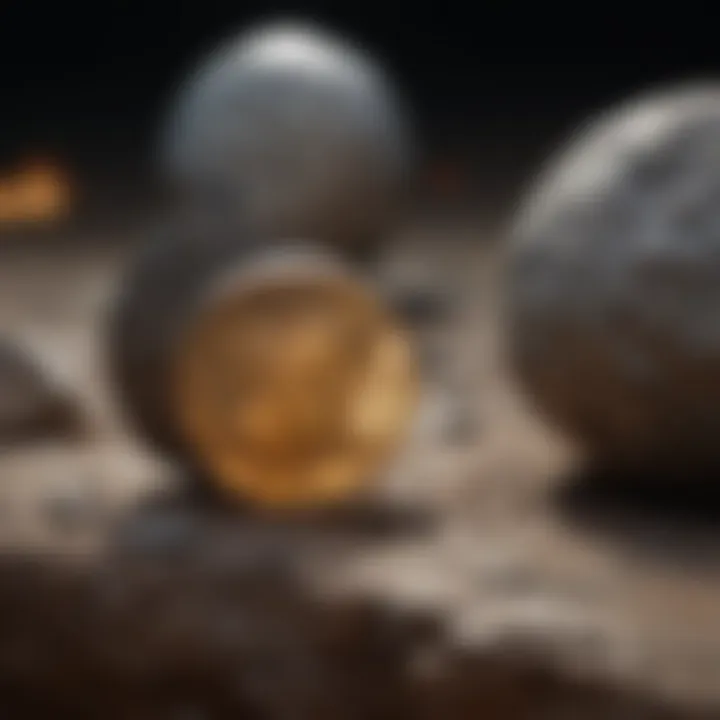
Practical Implications of Hardness Values
Hardness values aren’t merely numbers; they reveal a treasure trove of information about a material’s potential uses and limitations. For collectors, understanding the practical implications of these values can elevate their knowledge and appreciation of their specimens. A high hardness value often suggests that a material can withstand wear and tear better than softer alternatives. For instance, jade’s remarkable hardness makes it highly prized in jewelry and carvings.
On the other hand, lower hardness values can indicate materials that are more susceptible to damage. This can be crucial information for collectors who want to showcase their pieces but need to prepare for certain conditions that might degrade softer materials over time.
Beyond aesthetics, hardness data can also inform decisions in industrial applications. Manufacturers often seek materials with optimal hardness values for specific purposes—think about the cutting tools industry, where the hardness of various elements can drastically influence performance. Keeping hardness data in mind can aid collectors who want to cross over into commercial transactions, enabling them to better assess the market value of their pieces.
In summary, interpreting hardness data plays a crucial role in understanding material qualities, facilitating informed decisions in both personal collections and professional applications. By mastering comparative analyses and recognizing the practical implications of hardness values, one not only becomes a better collector but also gains a deeper insight into the fascinating world of materials.
Choosing the Right Hardness Test
When it comes to assessing material properties, selecting the appropriate hardness test is paramount. Each method has its own quirks, and what works well for one material might fall flat on another, potentially leading to inaccurate results. For rock and fossil collectors, this choice isn’t just academic; it tells you about the material's integrity and usability, which can affect valuation and desirability.
The importance of choosing wisely cannot be overstated. A wrong choice can mislead interpretations, affecting everything from material selection for projects to properly pricing collectible specimens. In essence, it can either make or break your understanding of the material at hand.
Factors to Consider
When selecting a hardness test, several key elements should be on your checklist:
- Material Type: Different materials respond uniquely to various hardness tests. For instance, metals may yield different results when subjected to Rockwell compared to Vickers tests. Rock and mineral hardness often require the Mohs scale for practical assessments.
- Sample Size: Not every test can accommodate every size. Some methods need larger sample slices, while others work just fine with tiny chips. Do consider your specimens.
- Surface Condition: The surface of your sample should also be in decent shape. Surface roughness can skew results, particularly in methods like Vickers and Brinell where indentation precision is key.
"Picking the wrong hardness test is like using a hammer when you need a scalpel. Precision matters, especially in material science."
- Desired Outcome: What exact data do you want from the test? Are you interested in comparative analysis or precise numeric values? Setting clear objectives will guide your choices.
- Environment: Finally, factors like temperature and humidity can affect readings. It’s often overlooked, but if you’re testing in a lab versus a field environment, outcomes can differ.
Testing Equipment Comparison
Comparing the equipment used in various hardness testing methods will provide deeper insights into the strengths and weaknesses of each test:
- Mohs Kit: This is a simple, portable solution perfect for collectors. Made up of ten reference minerals, it’s handy but subjective—results can vary based on user interpretation.
- Vickers Hardness Tester: This is more complex and suitable for lab environments. The diamond pyramid indenter makes it far more precise than most techniques. Yet, it’s typically more costly and requires a controlled setup.
- Brinell Tester: This method involves a hardened steel ball pressed into the material. While it’s suitable for larger materials, the calculations can become tedious when interpreting the results.
- Rockwell Tester: Fast and straightforward, it’s excellent for metals but may not distinguish finer details in softer materials. The simplicity is its charm as much as its limitation.
Choosing the right hardness test isn't just about personal preference. It reflects deeper evaluations necessary for material classification, particularly for those engaged in serious collecting. Unraveling the nuances of hardness tests equips collectors with a toolkit—one that enhances their appreciation and understanding of their geological treasures.
Common Mistakes in Hardness Testing
When it comes to hardness testing, accuracy isn’t just a nice-to-have; it’s a non-negotiable. Mistakes made during this process can lead to serious misjudgments about material properties, which in turn can affect decisions in both commercial and personal contexts. This section sheds light on some common pitfalls in hardness testing, particularly focused on improper technique and neglecting calibration. Understanding these errors not only aids in preventing them but also enhances your overall competency in assessments.
Improper Technique
A significant number of errors in hardness testing stem from improper technique. This can encompass a range of issues, from how the test is performed to the conditions under which it takes place. For example, when using the Vickers hardness test, if the indenter is not cleaned properly or if excessive force is applied, it can yield inaccurate readings. Similarly, when conducting the Mohs hardness test, one might not remember to use the right reference materials, or worse, might confuse them altogether.
It's also essential to maintain consistent pressure and duration while applying the indenter. If one were to, say, press too quickly or with too little force, the results might not accurately reflect the material's true hardness.
- Here are some critical aspects to focus on to avoid improper technique:
- Always ensure that the test surface is smooth and clean to allow for an accurate measurement.
- Use the appropriate indenter for the material being tested to avoid skewed data.
- Follow the specified load and dwell time for each test type to achieve consistency in results.
"A strong foundation built on the right techniques leads to meaningful results. Skimping on the fundamentals can only spell trouble down the road."
Neglecting Calibration
Calibration might seem like a boring, nitty-gritty task, but it is a linchpin in ensuring that tests yield trustworthy outcomes. Neglecting to calibrate testing equipment can produce an avalanche of inaccurate data, making a mockery of the test results. Most testing machines will come with a calibration schedule, and deviating from this can be detrimental, especially in fields where precision is paramount.
Even the best equipment isn’t immune to wear and tear. People often overlook factors like changes in humidity or temperature, which can impact the machine's performance. Suppose the Brinell tester has not been calibrated in a while; the readings could be off by a significant margin, which can have real-world implications, especially for collectors or manufacturers who rely on precise material assessments.
- To avoid this common mistake, consider the following:
- Implement a routine calibration schedule, following manufacturer’s guidelines closely.
- Conduct checks regularly, especially before important testing sessions.
- Document calibration results for future reference to ensure transparency and accountability.
With the right knowledge, the road to accurate hardness testing becomes clear, allowing enthusiasts and professionals to engage with materials more effectively.
Future Trends in Hardness Testing
In the ever-evolving landscape of material science, understanding future trends in hardness testing is crucial. As we move towards greater technological integration and efficiency, the ways in which hardness is measured and interpreted will continue to change. This section dives into the heart of what’s next in this vital area, spotlighting the benefits and considerations surrounding advancements and new applications in hardness testing.
Technological Advancements
Technology is driving a revolution in how hardness testing is approached. New methods are popping up like mushrooms after a rain, and they promise not only greater accuracy but also efficiency that can save both time and resources.
Firstly, digitalized methods are a significant leap forward. Traditional methods often require subjective interpretation of results, but advanced software now provides automated analysis. This reduces human error, making results more reliable than ever. Instruments such as digital durometers now integrate sensors that can feed data directly into databases, enabling real-time analysis and sharing of information.
Moreover, the development of micro and nano-hardness testing technology has opened up a previously inaccessible realm. Tests that can measure hardness at minute scales are hugely beneficial for industries working with thin films or small particles. This technology paves the way for applications in electronics and thin coatings, where understanding the material properties at a microscopic level is critical.
In the world of rock and mineral testing, portable hardness testers using ultrasonic techniques gain traction. They allow for testing in situ, bringing lab capabilities right to the field. This means collectors can get immediate results without having to send samples back to a laboratory for analysis.
Innovations in AI are also beginning to touch on this arena. Machine learning algorithms can analyze data trends across multiple tests, enabling predictive maintenance and helping identify potential issues in materials before they arise.
"The future of hardness testing is not just about measurement – it’s about intelligent measurement that leads to informed decision-making."
Emerging Applications
The future of hardness testing is not confined to just measuring the durability of metals or minerals; new applications are branching out into exciting territories.
One of the most intriguing areas is in the realm of biomaterials. As more industries lean towards sustainability, testing the hardness of natural materials, like plant-derived composites or biodegradable plastics, becomes increasingly relevant. These materials require precise hardness values to ensure they can withstand real-world conditions while still being eco-friendly.
Emerging fields like 3D printing are also seeing the integration of hardness testing. As additive manufacturing grows, assessing the integrity of the layers being printed is essential to ensure quality outcomes. This might involve innovative hardness testing methods to balance strength and flexibility, which is vital in aerospace or medical applications where failure is not an option.
Additionally, the art market is beginning to use hardness testing to verify the authenticity of historical artifacts and artworks. Assessments can help determine if a piece is indeed what it claims to be or if it has been tampered with. Given the prevalence of forgeries in the art world, the importance of accurate hardness testing cannot be overstated.
In summary, as we gaze into the future, it's clear that the world of hardness testing is set to burgeon. From technological advancements enhancing existing methods to entire new arenas opening up for application, collectors and professionals alike should keep their eyes peeled for developments that will inevitably enrich their understanding and practice of hardness testing.